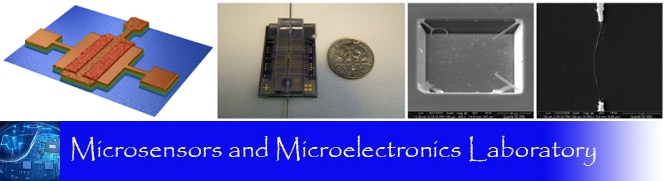
Research Interest
- Functional materials, nanomaterials, and polymer composites
- Sensors (physical, chemical, and biological sensors) and sensor materials
- Micro-/nano-electronics and semiconductor devices
- Micro/nanoelectromechanical systems (MEMS/NEMS)
- Fabrication technology at micro- and nano-scales
- Integration of sensors, electronics, and biomaterials
- Wearable and flexible electronics
|
Current Project: Polymer-Nanoparticle Composites as Cryogenic Dielectrics |
Polymer-based nanocomposites have attracted a lot of attention
lately, due to their enhanced or modified properties from the host
polymers. Our team is investigating nanoparticle-enhanced polymers, as
nanocomposites, for various applications. One particular area we are
exploring is polymer/nanoparticle dielectrics. Adding nanoparticles to
polymers not only can potentially strengthen their dielectric strength,
but also can introduce other interesting features such as optimized
chemical, mechanical, and thermal properties to the final material.
(Collaborator: Dr. Robert Krchnavek, Dr. Lei Yu, Dr. Behrad Koohbor) |
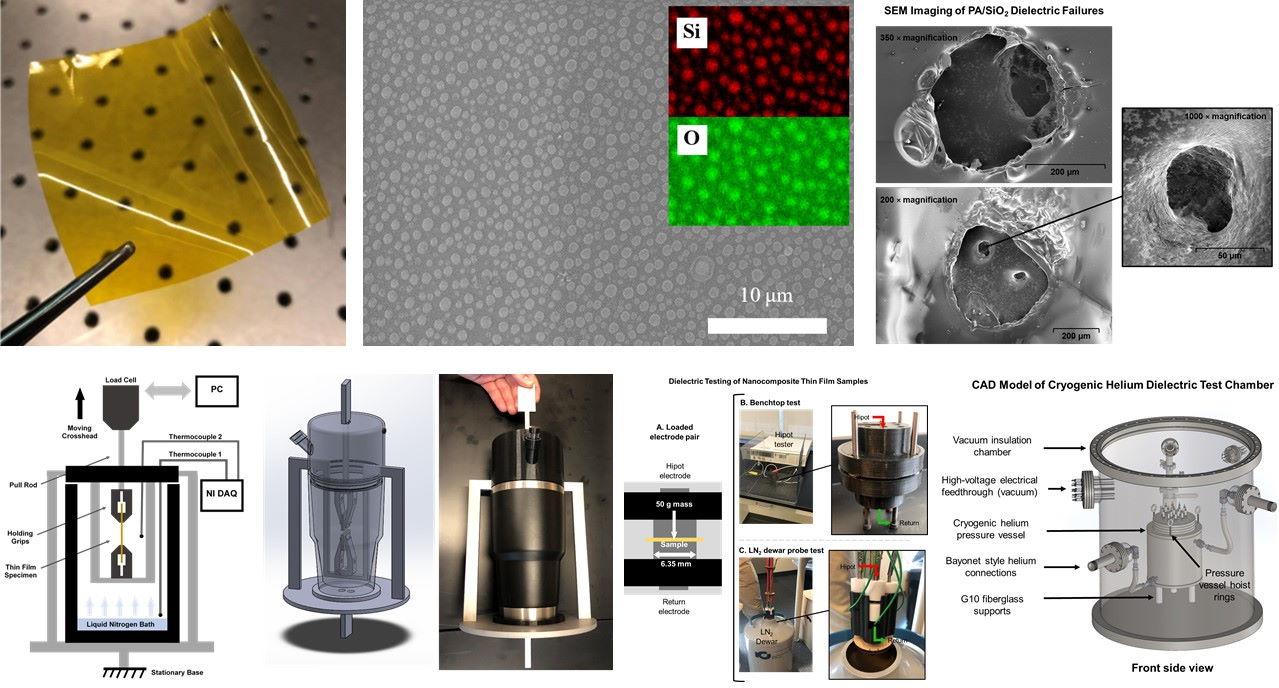 |
|
Current Project: Wearable Electronics and Systems | Wearable
electronics is a potential game changer for healthcare; it can
drastically transform the way patients are diagnosed and treated. We
aim to research and develop wearable systems that combine the
state-of-the-art sensor technology, modern electronics, and
biomaterials. Our objective is to create devices that are light weight,
highly flexible, and comfortable to wear. A key aspect we are exploring
is the integration of MEMS sensors into flexible, biocompatible
materials such as silk or protein materials. (Collaborators:
Dr. Robert Hirsh, Dr. F. Mac Haas, Dr. Jeffrey
Hettinger, Dr. Adarsh Gupta, Dr. Thomas Merrill) | 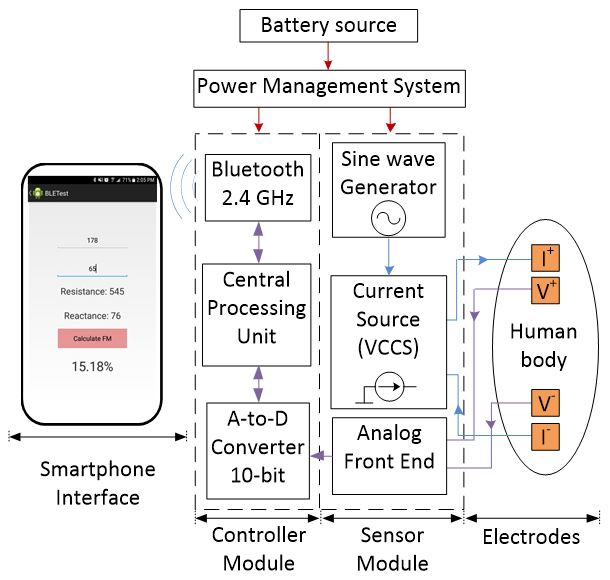
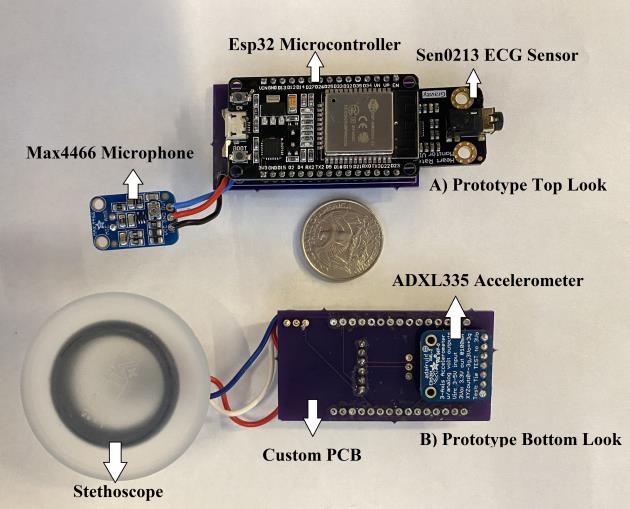 |
| Current Project: Nanomaterial-enabled Energy Harvesters | An
average human body produces a large amount of energy throughout the
day. Energy harvested from human body movement such as footfalls and
arm swings can produce continuous, stable energy to portable
electronics and implanted medical devices. For these applications, the
energy harvesters need to be light, small, inexpensive, and highly
portable. We are investigating novel wearable energy harvesters that
can be curved to human skin for electricity generation. One approach is
to integrate polyvinylidene fluoride (PVDF) nanofibers with
flexible substrates; the other one is to combine piezoelectric
nanoparticles into a host polymer. In the long run, these new devices
can continuously harvest vibrational energy from heartbeat or muscle
contraction for electrical use. This research targets the market of
healthcare systems. It will have tremendous impact on implanted medical
devices and the overall healthcare market. (Collaborator: Dr. Vince Beachley and Dr. Xiao Hu) |
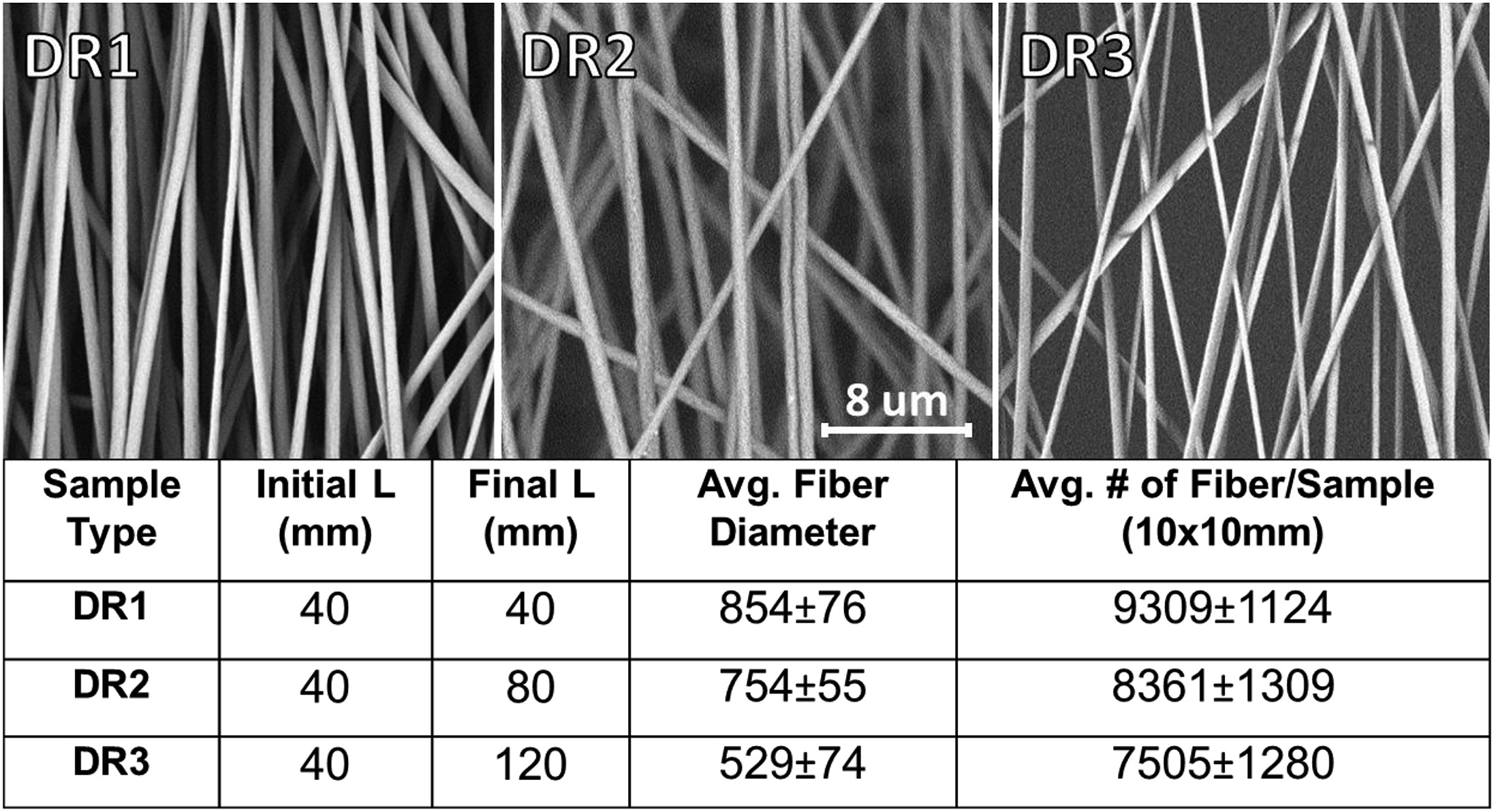
|
| Previous Project: Superhydrophobicity and Hydrophilicity Enabled by Micro/Nanotechnology | Inspired
by the “lotus leaf effect” and its related biological structures, the
research on superhydrophobic surfaces has attracted tremendous
attention in the past decade. Many surfaces in nature exhibit
superhydrophobic behaviors with contact angles greater than 150
degrees. Such surfaces typically possess unique properties. Because
many of these surfaces have well-arranged micro/nano-structures, the
creation of these surfaces can be achieved through
micro/nano-fabrication methods. The superhydrophobic surfaces are
finding applications in a wide range of areas, e.g. water-repellent
coatings, self-cleaning fabrics, and anti-icing films. We have applied
different methods to change the surface hydrophobicity of various
materials, resulting in surfaces with a wide range of wettability: from
superhydrophobic to superhydrophilic. | Pillar array to change the wettability of solid materials. The water contact angles range from 170 to 10 degrees |
| Previous Project: Microfluidic Devices with Integrated Carbon Nanotube (CNT) Sensor Arrays | The
innovations in microfluidics and microfluidic devices have given sensor
technology new meaning and new opportunities. For example, a
microfluidic exchange system superimposed on a sensor can actively
transport target analytes to the sensor surface, enabling the detection
of analytes at ultra-low concentrations. In our group, we investigate
the effective integration of nanomaterials, electronics and
nanosensors, and microfluidic structures into a lab-on-a-chip device.
The integrated lab-on-a-chip device is designed as a sensing platform
which has great potential for future expansion in various applications.
To create the sensors, we examine the selective deposition of
single-walled carbon nanotubes (SWNTs) with dielectrophoresis to obtain
aligned nanotubes in the forms of thin films, small bundles, and
individual nanotubes. These different results are achieved by changing
a number of parameters in the dielectrophoresis process and the SWNT
samples. The fabrication process is compatible with the traditional
microfabrication technology and has a high potential to be used in the
wafer-level fabrication in the future. | Lab-on-a-chip microfluidic system
conceptual design Microfluidic device with integrated CNT sensors
Aligned individual CNTs
Aligned CNTs between electrodes
Aligned graphene between
electrodes |
| Previous Project: Carbon Nanotube Electronics and Sensors | We
have used carbon nanotubes to create electronic devices such as
resistors, field-effectors, and digital logic devices on various
substrates. The high mobility of carbon nanotube enables the
development of faster electronic devices. These devices can also be
used for sensors, especially chemical and bio-sensors, based on their
high surface-to-volume ratio. They demonstrate higher performance
compared with their counterparts made on silicon or other nanomaterials. |
Surface profile of a CNT field-effect transistor (FET)
Enzyme biosensor
using ion-sensitive FET (ISFET)
CNT
digital inverters
CNT
electronic devices on plastic
CNT biosensors on plastic
CNT FET structures and
materials
Testing diagram for CNT ISFET biosensors |
| Previous Project: Novel Micro/Nano Fabrication Methods | We
are interested in all kinds of micro/nano fabrication methods and how
these methods can be integrated into one process flow to fabricate
devices. In the past, we have explored various approaches for both
top-down and bottom-up fabrication processes, including hot embossing,
layer-by-layer self-assembly, electron-beam lithography, and
dielectrophoresis. We have also explored various methods for substrate
bonding and device assembly. |
| Previous Project: Physical Sensors and Actuators on Polymers | We
have used polymer materials (PMMA) to make physical MEMS devices,
including all-polymer actuators, accelerometers, and tunning magnetic
sensors. Novel fabrication methods such as hot embossing are used to
produce polymer structures at the microscale. We have developed the
expertise of theoretical analysis, modeling (by ANSYS and MATLAB),
fabrication, and device testing of mechanical sensors. (Advisor: Dr.
Tianhong Cui; Collaborators: Dr. Jing Wang, Dr. Yongjun Zhao) | Design of a comb drive for an accelerometer
Fabricated polymer
comb drive accelerometer
Tunneling
tip on polymer
Polymer magnetic sensor
Journal cover
paper |
|
|